Introduction
The main purpose of shifting our
energy consumption over to alternative energy sources like solar and
wind is of
course to avert Global Warming by decarbonizing the atmosphere.
We all know that completely decarbonizing our civilization's energy
supply is a huge task - it means we need to replace essentially all the oil used by vehicles as well
as the coal and gas used for electrical generation. This article is a
thought experiment that attempts to illustrate just how how big that
task is.
The carbon-free energy sources available for consideration include
hydro, wind, solar, tidal, geothermal and the carbon-free source
everyone loves to hate, nuclear power. Most good hydro sites are
already exploited, solar output is still too small and tidal
and geothermal power are still too new to make
convincing cases as global power sources. In the interests of
avoiding controversy, I won't use nuclear power either.
Wind,
on the other hand, is a
well-understood technology with reasonable output, and it appears
to be scaling pretty well. So in
this article I'm going to examine what it would take to completely
decarbonize modern industrial civilization using only wind power.
The Numbers
So, how much wind power would it take to do the job?
First,
I’ll set out my assumptions:
- We
may need gas turbines as peaking plants, so I propose to leave 10% of
the fossil fuel use intact for that purpose and replace the other 90%
of the oil, gas and coal we currently use with electricity from wind.
- I
propose to do it by 2050, giving us a nice generous 40 years to “git
‘er done”.
- The
requirement for primary energy would rise by 1% per year – this is less
than half the last 45 year average of 2.5%, but we’ll give up the other
1.5% per year to efficiency improvements and conservation.
- Since
we’re replacing thermal power with wind electricity, we get a 60%
improvement in energy converted to work, meaning we only need to
replace 40% of the fossil BTUs.
- We'll
do it all with 5 MW turbines that run at an average capacity factor of
23%
Now
let's dive into the calculations:
The
world currently uses 9800 million tonnes of oil equivalent (mtoe) per
year of fossil fuels. A 1% growth rate puts the 2050 usage at 14,750
mtoe.
Keeping 10% of that usage intact means we have to replace the work done
by 13,275 mtoe.
Applying our 40% conversion factor, we will need to replace about 5300
mtoe.
According to BP’s Statistical Review of World Energy 2010 (the source
for all the data here) the conversion factor they use for electricity
is 12,000,000 MWh per mtoe. So we will need to be able to generate need
63,600,000,000 MWh of electricity from wind in 2050.
A 5 MW turbine running at 23% capacity produces about 10,000 MWh in a
year
In 2050 therefore, we would need to have a total of 6,300,000 5MW turbines running
world-wide.
If we installed the same number of them every year, we would need to
install 157,500 turbines, or 787.5 GW of capacity every year to reach
the required capacity by 2050.
Of course, 40 years is beyond the life cycle of a turbine. Assuming a
20 year life cycle, we will need to replace all the 20-year-old
turbines at the same time as we’re installing the new ones. So by 2050
we’d be re-installing 5% of the operating plant each year, or 315,000
turbines a year, which we would need to continue forever.
That means installing 1575 GW of new capacity every year.
1575 GW of capacity requires 315,000 turbines every year, which
averages out to the manufacture and installation of one new 5 MW turbine every two minutes -
forever.
Last year we installed 37.5 GW. We will need to maintain 40 times that
installation essentially forever to get the job done.
The Cost
As a way of clarifying the scale of the task in my own mind, I decided
to see what the installation rates and costs were for this model.
I use the same basic assumptions here as above: the goal is to replace
90% of fossil fuels with wind power, keeping hydro and nuclear
contributions intact. It's worth remembering that even though I'm using
wind power in this example, the technology doesn't matter - it could
include CSP, PV, tidal, hydro in any mix. The important thing is that
the model replaces 90% of all fossil fuels (including gasoline, diesel
fuel, aviation fuel, natural gas and coal) with electricity. For the
purposes of this model, the only difference between the various
renewable generating technologies would be capital cost and life cycle.
Using one technology like wind is a simplification technique to make
the model manageable.
Since the build-out rate is chosen to reach the target by 2050, the
logistical differences between various renewable technologies aren't
considered, though they would obviously be a factor in real life.
For this analysis, instead of assuming a simple linear build-out I
approximated a logistical function. The build rate starts where it is
today, ramps very rapidly to achieve the majority of the installations
in the middle period from 2025 to 2040, then drops off as the capacity
requirement approaches saturation. The logistical curve isn't built
mathematically, there is no point to that for such a hypothetical
exercise. Instead it was constructed by hand in Excel to fit the curve
between the known start point (today) and the desired endpoint (32
million MW of wind capacity in 2050).
The first graph shows the growth of cumulative generating capacity over
the period of the program (2010 to 2050) plus an additional 10 years
beyond that.
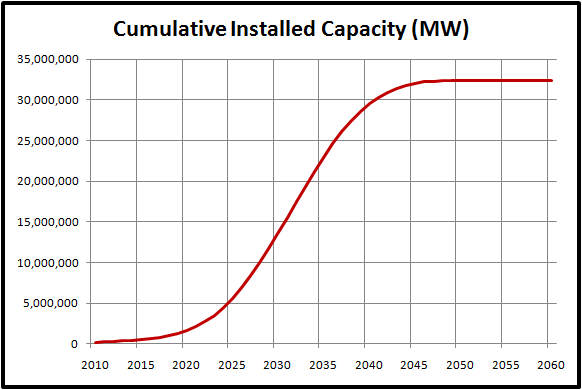
The next graph shows the installation of new turbine capacity over time.
It peaks in 2033 at a bit under 2 million megawatts of capacity, or
400,000 turbines.
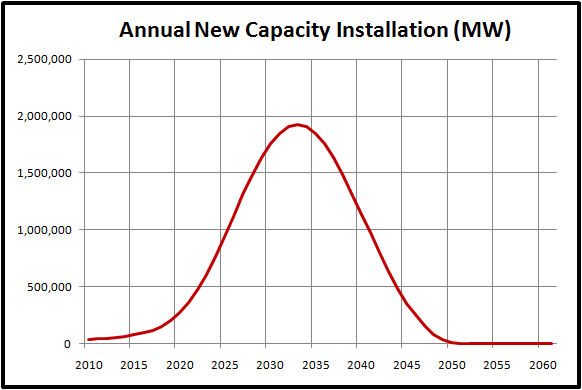
The installation of new capacity isn't the only installation activity,
though. As the program goes into its second half, the turbines that
were installed 20 years before are coming to the end of their life
cycle and need to be replaced. While some of the old materials will be
re-used, these replacements count as new construction. The difference
is that this activity remains constant even after the goal of 32
million MW has been reached. In fact, it continues in perpetuity. No
new capacity is added by these installations, their purpose is simply
to maintain the existing generating capacity. At the end of the program
40 years hence there are two end-of-life replacement cycles under way -
the one for the new turbines that were
installed 20 years before, and the one for the replacement turbines also installed 20
years ago.
When we combine the new installations with the life cycle replacements
we get the following curve:
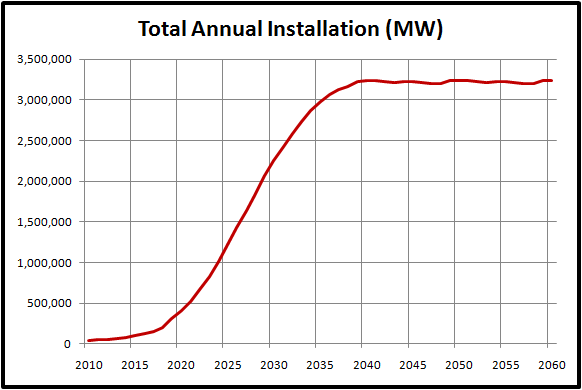
How much is all this going to cost? The cost estimates I used
are European, from EWEA. They translate to $1.68/watt in current US
dollars.
I factored in an ongoing 1% per year drop in costs that brings the cost
down to $1.00/watt by 2060.
The program cost peaks in 2040 at about $4 Trillion annually. The total
program cost to 2050 is $100 Trillion, with ongoing life cycle
replacement costs of about $3T to $3.5T annually after that. These are
just the capital cost of turbine manufacture and installation, they
don't include changes to the grid required to accommodate variability
or to permit the integration of such a high proportion (60%+) of highly
variable wind power.
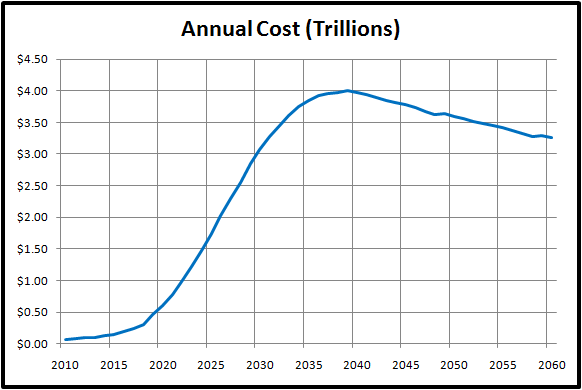
Next I considered how such costs would impact spending in other areas
of the global economy. One way to get a feel for this is to consider
the costs as a proportion of Gross
Capital Formation or GCF. GCF is the proportion of GDP used to
produce assets such as machinery. Global GDP is currently around $60T
annually, but GCF accounts for only 22% of that (~$13.5T) - the rest is
spent on services.
The impact of the program on the capital portion of the global economy
becomes very significant around 2025, rising to the level of a severe
impact in 2030, and remaining severe from then on. Since the 25% or
more of GCF consumed by this program is not available for other
manufacturing investment this is bound to cause a lot of political
resistance.
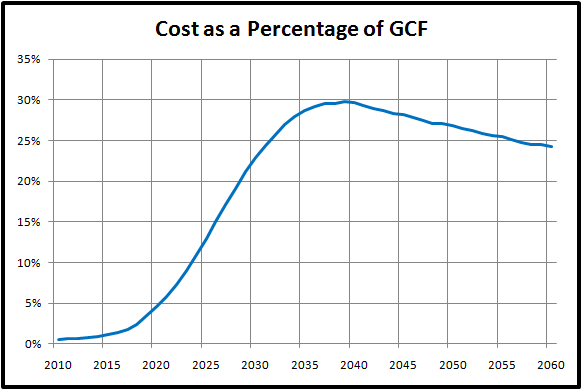
Conclusion
Replacing 90% of our fossil fuel use with electricity from wind power
is an overwhelming task from a number of perspectives: material
requirements, manufacturing and installation capacity development, and
the high financial expenditures required immediately and maintained in
perpetuity.
As I mentioned above, this thumbnail sketch doesn't include essential
grid upgrades, and it also ignores the problems inherent in switching
close to a billion cars from gas to electricity. Other issues that are
not addressed include aviation and the military, both of which have
unique requirements for combustible fuels; the question of how to
promote the abandonment of sunk costs in functioning fossil-fuel
infrastructure; and the social and political resistance to over 6
million wind turbines dotting the global landscape.
Paul Chefurka
November 17, 2010
|